RESEARCH: PHOTOMETRY
CURRENT RESEARCH INTERESTS AND PROJECTS AT FLORIDA SPACE INSTITUTE
The Photometry Group at Florida Space Institute is actively engaged in predicting, observing, and analyzing stellar occultations by small bodies in the outer solar system. Each semester, we compile a list of the best occultation opportunities and collaborate with observers worldwide to acquire images. We welcome partnerships with both professional and amateur astronomers at any time; please contact us if you are interested in collaborating.
Stellar occultations provide high-resolution, sub-kilometer measurements of a small body’s limb, significantly improving our understanding of its orbit. These observations have enabled key studies, such as i) Pluto’s atmosphere follow-up observations, which provided valuable insights into how Pluto’s atmosphere evolves over time; ii) Topographical features and ring systems: Stellar occultations have helped identify surface features and revealed four ring systems around some small bodies; iii) Objects shape an size: dozens of small bodies have been detected and their physical properties constrained by using this technique. Most of these important results come from ground-based observations, and we continue to explore new collaborations to further enhance our research.
WHAT IS PHOTOMETRY?
Photometry, a portion of Solar System Astronomy, is essential for understanding the physical properties of celestial bodies, such as their size, composition, and surface features, by measuring the intensity of light they reflect. This data provides crucial insights into the dynamics, evolution, and potential habitability of planets, moons, and other solar system objects.
To understand the research we pursue at Florida Space Institute in the context of Photometry, it is important to know the fundamentals of it.
Photometry is an essential tool in space science for quantifying the brightness of celestial objects. It involves measuring the intensity of light received from stars or other astronomical bodies and translating this data into meaningful values such as magnitudes. This process can be divided into two primary methods: differential photometry and absolute photometry.
In differential photometry, the brightness of the target object is compared with that of nearby comparison stars. The difference in brightness is calculated by comparing the Analog-to-Digital Unit (ADU) counts from the target and the comparison stars. This method allows astronomers to determine the relative brightness of an object with respect to a known standard. In contrast, absolute photometry requires no comparison star and involves measuring the object’s brightness in absolute terms by analyzing its ADU count. This method is more complex, as it necessitates careful calibration of instrumental systems and more elaborate data processing to ensure accuracy.
Photometric observations must account for the varying sensitivity of different filters, detectors, and observing systems. The UBV system (Ultraviolet, Blue, and Visual) was established by Johnson and Morgan as a standard photometric system using wide-band filters and photomultipliers. This system, later expanded to the Johnson-Kron-Cousins system with the addition of red and infrared filters, serves as a benchmark for observers worldwide. Calibration of observational data is performed by comparing observed magnitudes with cataloged magnitudes of standard stars. Transformation coefficients are used to correct for differences between the observer’s equipment and the standard system, enabling more accurate comparisons.
To conduct photometry, a point source like a star is imaged on a CCD chip, where its light is spread across several pixels due to atmospheric turbulence and optical diffraction. The Point Spread Function (PSF) describes this spread, typically approximated by a Gaussian curve. For crowded fields, such as globular clusters, the PSF method is employed, where the spread of light from multiple bright stars is used to model the star’s profile. In contrast, aperture photometry sums the light from within a synthetic aperture centered on the object. This method works well for isolated stars but may encounter issues in crowded fields or with faint stars, where background light contamination becomes significant.
To address sky background contamination, an annular region around the star is used to estimate and subtract the background light, ensuring more accurate measurements of the object’s light. This technique is essential for removing the influence of the night sky’s light on the measured signal, a critical step for accurate photometric data.
Once the ADU count for the object has been determined, the next step is to calculate its instrumental magnitude, which provides a preliminary brightness value. To convert this into a meaningful, absolute magnitude, several correction factors must be applied. The first-order extinction coefficient accounts for the absorption and scattering of light as it passes through the Earth’s atmosphere. This effect is more pronounced for shorter wavelengths, which are absorbed more than longer wavelengths. The air-mass, a measure of the amount of atmosphere the light passes through, plays a key role in determining the extinction effect. Observers use known stars at varying air-masses to calculate the extinction coefficient, which corrects for atmospheric effects and provides a more accurate magnitude for the object.
In addition to first-order extinction, second-order extinction may be necessary for precise work, particularly in the visual and blue wavelengths. This correction accounts for color-dependent extinction effects and is typically only relevant for very high-precision measurements. However, for most photometric work, the first-order extinction coefficient suffices.
To further improve accuracy, transformation coefficients are applied to account for differences in the sensitivity of different photometric systems. These coefficients are derived by comparing the instrumental magnitudes of standard stars with their cataloged values, and correcting for any discrepancies caused by the observer’s equipment. The transformation process ensures that the data can be aligned with a standard photometric system, allowing comparisons across different observations and telescopes.
Finally, zero points are used to account for offsets in the measurement system, typically reflecting the sensitivity of the instrument used for observation. The zero point is derived by analyzing the instrumental magnitudes of standard stars and adjusting for any systematic errors. While the zero point tends to remain stable during an observing run, small fluctuations can indicate potential issues with the equipment or observational conditions, such as changes in sky transparency.
In conclusion, photometry involves a series of detailed steps to ensure accurate measurements of the brightness of celestial objects. It requires careful calibration of instruments, correction for atmospheric and color-dependent effects, and the use of standard systems to compare observations. By applying these methods, astronomers can achieve high-precision measurements of the light emitted by stars and other astronomical bodies, providing valuable insights into their physical properties, distances, and behavior across the cosmos.
*This information was sourced from the pdf attached here, source material credits to the original author.
Here are some of the main components we use research in Photometry to further understand celestial phenomena
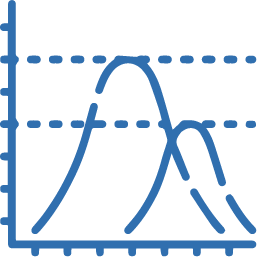
LIGHT CURVE ANALYSIS
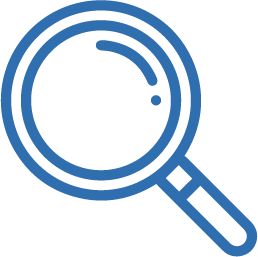
DATA REDUCTION AND MODELING
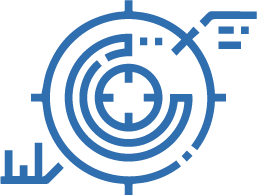
CALIBRATIONS
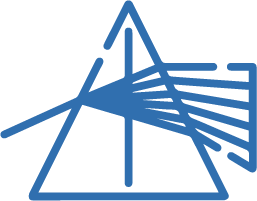
SPECTRAL CLASSIFICATIONS
The video shows an occultation by the Centaur (10199) Chariklo on 2017 June 22. Observation was made with a Raptor Merlin EM247 CCD camera attached to a 50-cm portable Alt-Az telescope. Exposure time was 80 ms (data rate was about 12.5 Hz). Observation was made by Mike Kretlow (IOTA-ES: International Occultation Timing Association, European Section) at Onduruquea Guestfarm in Namibia., kindly supported by the Lucky Star ERC project (Observatory Paris-Meudon, PI Bruno Sicardy)